Research
Type of research
Molecular and cellular biology in context of adipose tissue physiology.
Main research areas
The maintenance of our body weight is determined by a system able to precisely match food intake with energy expenditure. In this context, two tissues are of utmost importance. The most abundant one is white adipose tissue, which stores excessive energy in form of triglycerides and provides free fatty acids to other organs when energy is needed. A more scarce organ is brown adipose tissue, which dissipates stored energy in form of heat in a process called adaptive non-shivering thermogenesis.
Brown adipose tissue
The main function of brown adipose tissue is to protect newborns and small rodents from cold. This is enabled by presence of uncoupling protein 1 (UCP1) on the inner mitochondrial membrane of brown adipocytes, which uncouples mitochondrial respiratory chain from ATP synthesis and the generated electrochemical gradient is released in form of heat. Brown adipocytes contribute to the maintenance of body temperature homeostasis during both chronic and long-term cold exposure. Recently, it was shown that active brown fat is present also in adult humans, most frequently in supraclavicular, paravertebral and deep neck region and it responds to cold as well as to administration of a specific B3-adrenoreceptor agonist. It was estimated that even small amounts of active brown adipose tissue could lead to a significant increase in basal energy expenditure thus promoting weight loss and preventing development of metabolic disease. Activation of brown adipose tissue function therefore might be a promising strategy for increasing energy expenditure and fighting the global obesity epidemics. We have recently shown that mature white adipocytes have the potential to interconvert between white and brown phenotype in response to environmental temperature change. This means that the ratio of lipid-storing to lipid-burning cells can vary within adipose depot without the need for de novo differentiation.
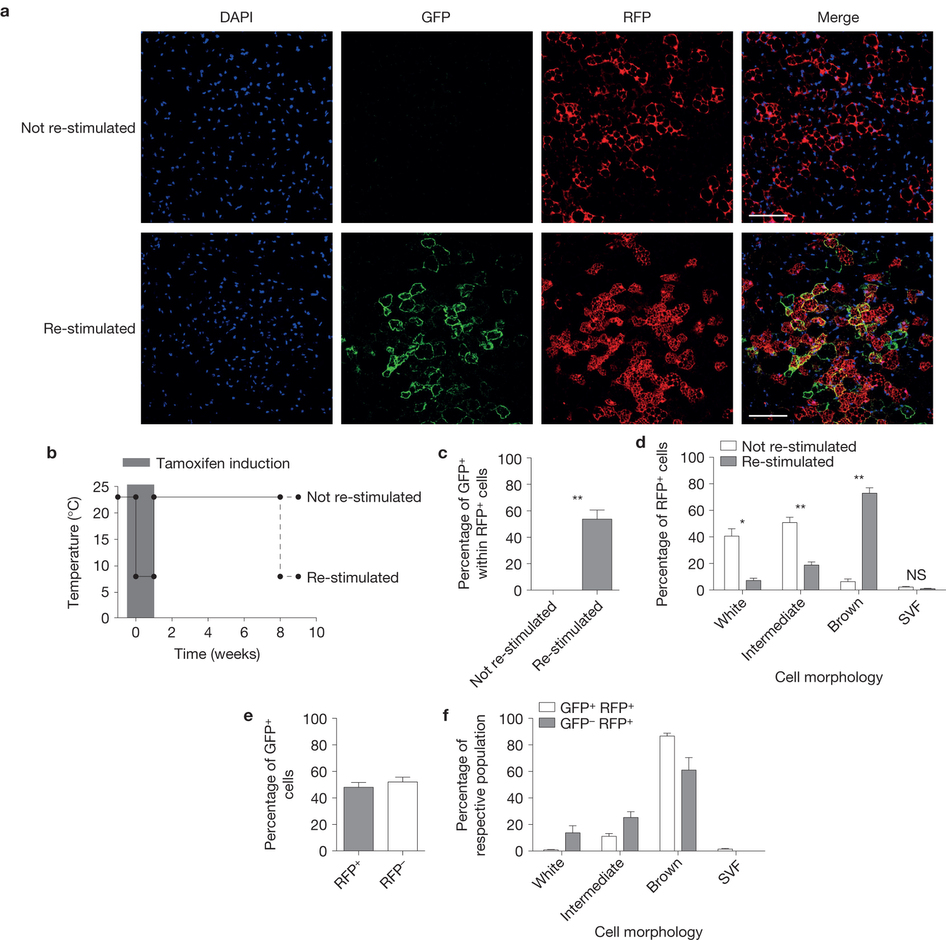
The lab studies in detail the molecular mechanisms of adipocyte conversion and is analyzing several novel pathways that regulate brown adipocyte formation and activity.
White adipose tissue
White adipose tissue has long been considered to be a passive storage of lipids, mechanic and thermal isolation. Discovery of leptin more than 20 years ago brought a novel insight into understanding of its function. Currently, adipose tissue is considered a highly dynamic multifunctional organ producing and releasing a whole spectrum of biologically active substances, collectively termed adipokines. Several adipocyte-derived molecules that influence whole-body energy homeostasis and regulate processes such as blood pressure, immune function and angiogenesis were identified to date. Adipose tissue is made up from a variety of different cell types and mature adipocytes constitute only a small portion of the adipose tissue cell content. Expansion of adipose tissue mass in response to excessive food intake is achieved through hypertrophy and hyperplasia. Whereas adipocyte hypertrophy (increase in size) is strongly associated with insulin resistance and local tissue inflammation, hyperplasia (increase in number) is associated with improved insulin sensitivity. In addition, changes in adipocyte size influence also the adipokine profile. Therefore, it is clear, that proper function of adipose tissue largely depends on the balance between adipocyte formation and hypertrophy.
We have identified several pathways controlling adipocyte formation.
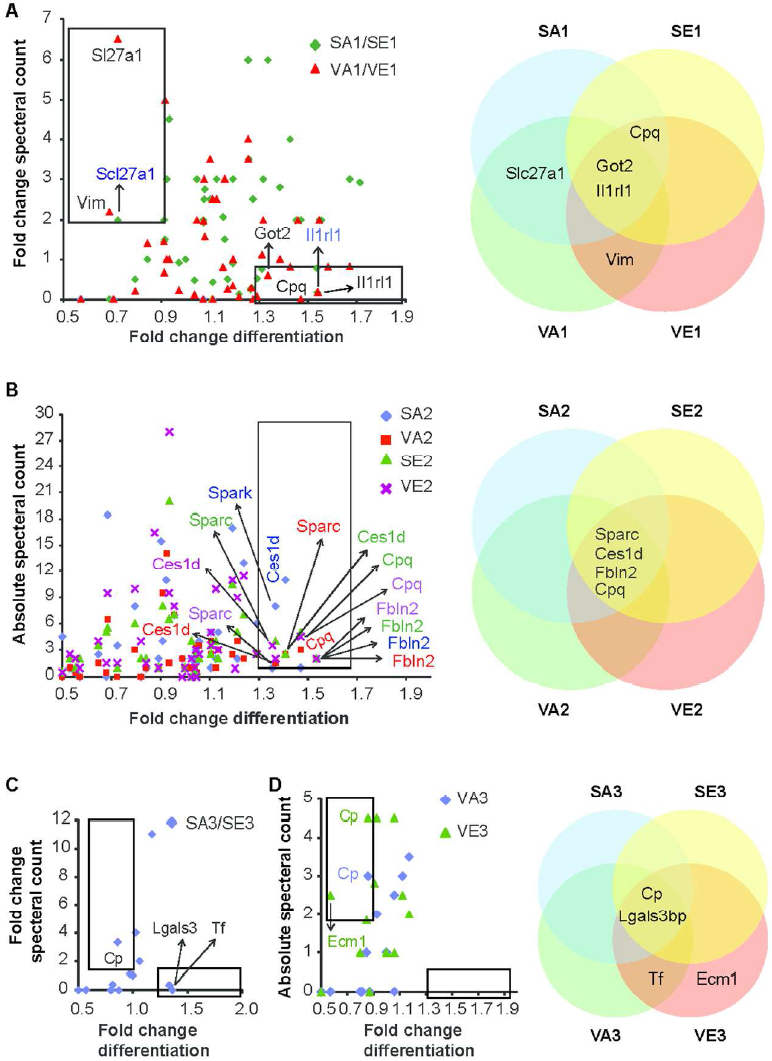
The lab is currently working to understand the molecular mechanisms and physiological implications of preadipocyte proliferation, commitment and differentiation.
Maternal overnutrition
Obesity has long been identified as a global epidemic with major health implications such as diabetes, cardiovascular disease and cancer. Maternal overnutrition and maternal obesity are significant health issues in industrial countries and are known risk factors for the development of obesity and related disorders in the offspring. The wide accessibility of “junk” food in recent years is one of the major causes of obesity. An excess of nutrients during fetal life not only has immediate effects on the fetus but also has long term effects on adult health.
Our interest is how maternal overnutrition can affect the development and function of the central nervous system circuits that regulate reward-related behaviors and the increased risk to develop obesity and related metabolic disorders in the offspring. Further, we seek to understand the underlying epigenetic mechanisms leading to heritability of addiction-like behaviors and obesity following maternal overnutrition.